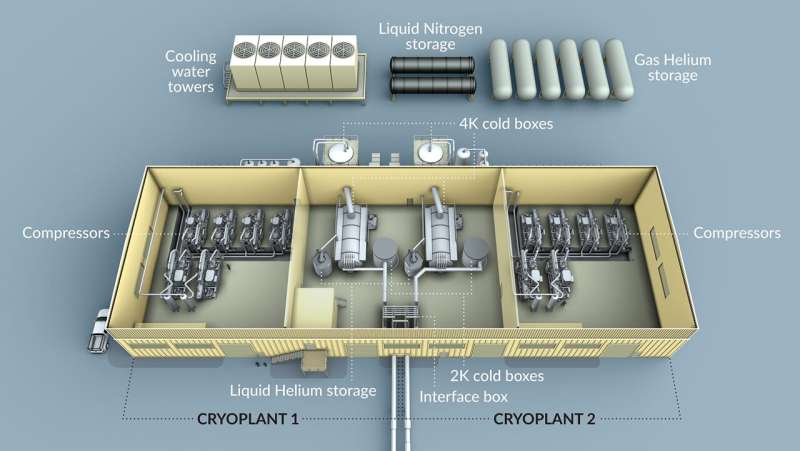
Schematic of the LCLS-II cryoplant. Credit: Greg Stewart/SLAC National Accelerator Laboratory
Today, it only takes an hour and a half to make a superconducting particle accelerator at the Department of Energy’s SLAC National Accelerator Laboratory, which is cooler than outer space.
“Now you click a button and the device goes from 4.5 K to 2 K,” said Eric Fove, director of the Cryogenic team at SLAC.
While the process is now fully automated, getting this accelerator, called LCLS-II, to 2 Kelvin, or minus 456 degrees Fahrenheit, took six years of designing, building, installing and starting a complex system.
The original LCLS, or Linac Coherent Light Source, accelerated electrons to produce the X-rays used in atom and molecule screening experiments. LCLS-II will run concurrently with LCLS. However, unlike LCLS, which use copper parts in Room temperature To speed up electrons, the LCLS-II upgrade uses chromium superconducting units. These frozen units transmit electrons with energy more efficiently, which will help generate more powerful X-ray pulses to expand experimental possibilities across domains.
But while LCLS can operate at room temperature, LCLS-II must be cooled to 2 Kelvin, 4 degrees Fahrenheit above absolute zero, to become superconducting.
This means that SLAC needs a team to focus on the cool stuff.
Assemble a team to assemble cooling plants
Before the LCLS-II cooled down, there was no dedicated cryogenics group at SLAC.
“The biggest challenge we had was that this was the first time we had done this with a new team,” Fove said.
The LCLS-II Cryogenic team, now made up of 20 operators and engineers, formed in 2016 at SLAC to build the facility that cools the accelerator: a cryogenic plant.
“This is a complex system with many subsystems working in tandem,” said Viswanath Ravindranath, chief cryogenic process engineer at LCLS-II.
SLAC worked closely with engineers from the Department of Energy’s Fermi National Accelerator Laboratory and the Jefferson National Accelerator Facility as well as leading cryogenic companies to design and procure materials for permafrost.
“This collaboration allowed the LCLS-II project to take advantage of the best cryogenic resources within DOE laboratories and elsewhere,” Fove said.
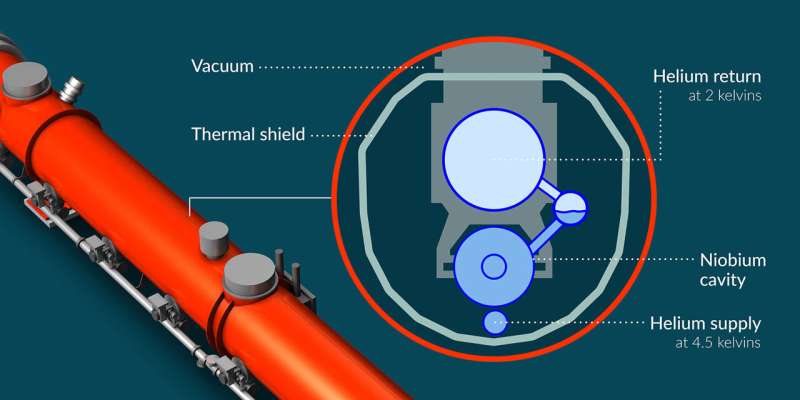
A cross section of the LCLS-II accelerator showing where liquid and gaseous helium flows in and out of the system. Credit: Greg Stewart/SLAC National Accelerator Laboratory
The seedlings are filled with cooling helium, which is cooled and then pumped to LCLS-II. While every other element freezes below 4 K, helium can remain liquid, and at 2 K, helium becomes super-liquid, meaning that it flows without viscosity. This fact, and superfluid helium’s ability to conduct heat better than any other known material, makes it the ideal coolant for superconducting accelerator cooling.
Before cooling begins, trailers stacked with sausage-shaped tanks deliver gaseous helium at ambient temperature (about 300 K) to external storage tanks in the cooler. A cryoplant requires a total of four metric tons of helium.
But this helium arrives impure. Any impurities will eventually cause the system to freeze and shut down, so first purifiers must trap any unwanted moisture or gases, such as nitrogen, to achieve 99.999% of helium.
After purification, the compressors raise the pressure of helium. The pressure and temperature of the gas are coupled: as the pressure decreases, the temperature also decreases. So, while later useful, this incidentally raises the temperature of helium to 370 K.
After compression, five large towers containing cooling water are used to reduce the temperature of helium again to 300 K. The gas then enters the 4K cold box in the cryoplant, which is a very complex giant helium refrigerator.
in the cold box liquid nitrogen Running 77 K knocks helium from 300 K to 80 K in a heat exchanger. In this device, warm helium gas and cooler liquid nitrogen travel in opposite directions while a thin metal plate separates them, transferring heat through the plate from helium to nitrogen. The plant uses 20 metric tons of liquid nitrogen each day.
The helium then passes through an assembly of four turbine extenders. Now the initial gas compression step pays off: turbine expanders expand the high-pressure gas, lowering its pressure enough to bring helium to 5.5 K.
However, helium has more expansion before it can leave the cold box. It goes through a valve that has a low pressure on the other side. This lower pressure causes the gas to expand, lowering its pressure and lowering its temperature to 4.5 K (hence the name 4K cooler), where it turns into a liquid.
This liquid helium is then sent through tubes to the freezing units of the accelerator, where the device cools down to 4.5 K.
Once the 4K cold box turned on, the Cryogenic team took one week to cool the LCLS-II from room temperature to 4.5 K, which it first reached on March 28, 2022. But that’s not cold enough!
still cooler
To reach 2 K, the 4.5 K helium undergoes another (final) expansion through a valve in the accelerator-freezing units. Again, the lower pressure on the other side of the valve causes the helium pressure to drop. This cools helium to a target temperature of 2 K.
Creating a low pressure inside the freezer unit is an achievement in itself.
“The magic happens when it goes through this valve, but only because we have a set of cold compressors that keep the pressure in the refrigeration unit at a very low pressure,” Fove said. This group of five compressors positioned after the valve creates the hub the pressure Variation on both sides of the valve.
After months of operating and configuring this cooling system, the LCLS-II finally reached 2 K on April 15.
“It was all possible because of all the hard work over the years from so many smart and dedicated people,” said Swapnil Shrishrimal, operations and controls engineer at LCLS-II. “Being a young team, as well as a young team, we are very proud of the system we have commissioned.”
When the electron beam is on and is accelerated by the freezing units, the 2 K helium will absorb heat from the accelerator, boil, and turn back into a gas. This gas is injected back into the 4K cold box to help cool the warmer helium.
“We don’t want to waste cooling capacity, so we try to recover as much of it as possible,” Ravindranath said. The system recycles helium, which is expensive, although necessary for long-term operation.
The Cryogenic team has already built two cryogenic plants, which share the building, but LCLS-II uses only one. The refrigerated second station will support the planned upgrades to the LCLS-II. When both cryogenic plants are in operation, they will use approximately 10 megawatts of electrical power.
Only four other cryogenic plants in the United States cool this much helium to 2 K. The Thomas Jefferson National Accelerators Facility and Fermi National Accelerators Laboratory, both of which house cryogenic plants of the same size, supported SLAC’s design and equipment procurement. SLAC has collaborated with Oak Ridge National Laboratory, Brookhaven National Laboratory, and CERN as well.
“Our years of experience and support from our partner labs have allowed us to do just that,” said Shrichermal. Fauve also credits the team’s success with thorough planning and dedication. The entire cryogenic team remained on site during the pandemic to continue reviving the plant.
“Even when SLAC was closed, if you were in a cryoplant you wouldn’t be able to tell the difference before and during COVID,” Fave said, except for masks and social distancing of course.
LCLS-II is expected to produce its first X-rays early next year. The Cryogenic team feel confident that they will continue to operate their highly complex refrigerator with ease.
“It’s a beautiful and easy process now because everything is automated,” said Shrichermal.
Superconducting X-ray lasers reach operating temperature cooler than outer space
the quote: Frightening Helium Cooling Particle Accelerator Flight (2022, August 31) Retrieved September 1, 2022 from https://phys.org/news/2022-08-helium-chilling-journey-cool-particle.html
This document is subject to copyright. Notwithstanding any fair dealing for the purpose of private study or research, no part may be reproduced without written permission. The content is provided for informational purposes only.